Bone regeneration is an advancing field of research
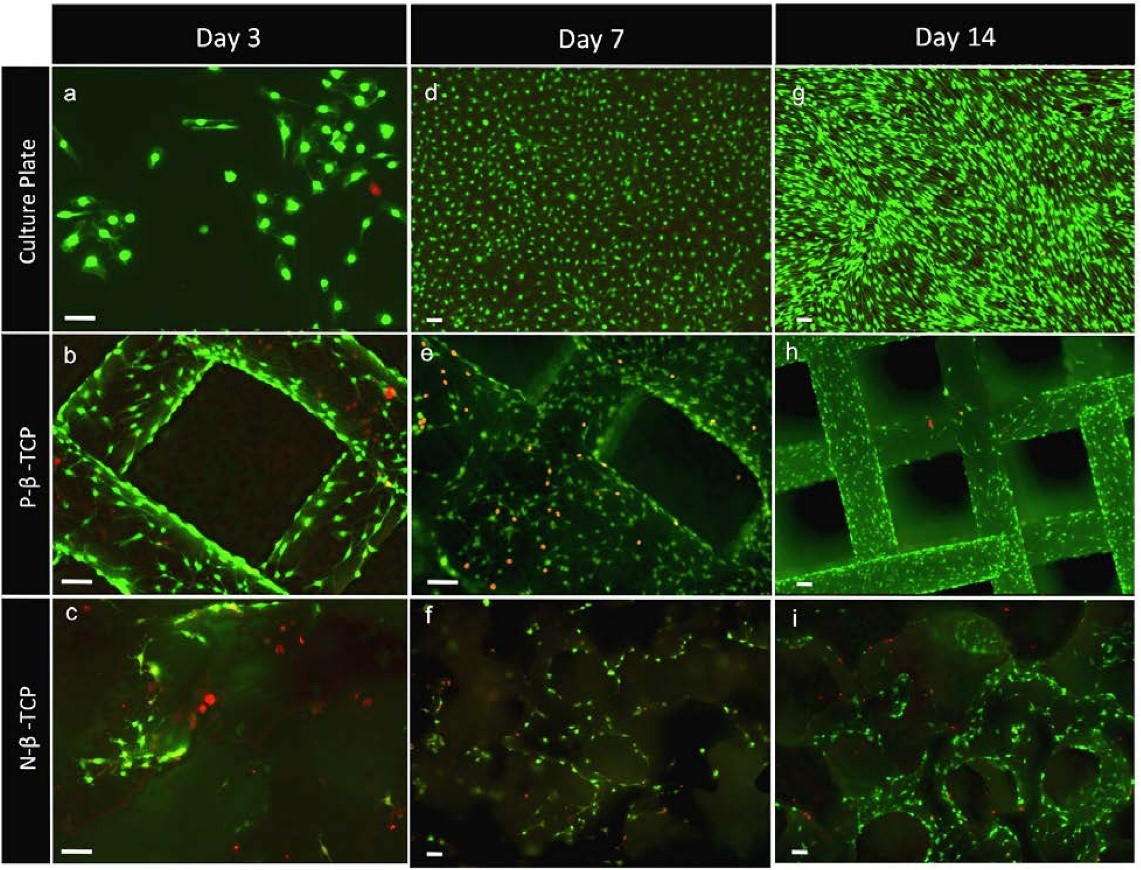
Bone regeneration is an advancing field of research
Unlike the structure and hierarchy of bone tissues, the process of bone regeneration remains an enigma and an intense area of research. Rightfully so, our bones stand as silent sentinels, adapting and responding to the stresses and strains imposed by daily life.
Today, it is well known that bone is formed in high-strain areas and removed in low-strain areas. Just like our skin, our skeleton is constantly remodeling and rejuvenating. However, bones are not impervious to disease or indestructible.
Periodontitis, osteonecrosis, osteoporosis and severe injuries can lead to bone loss and defects that extend beyond the innate ability of our bones to heal and recover. But when disease or injury does strike, researchers and clinicians can now look to enhance and support the remarkable rejuvenating ability of our bones. How? By breaking free of the traditional cell cultures and experimenting with 3D microenvironments using printed scaffolds that mimic natural bone.
Why is bone research needed?
Bone research is building upon the core and innate strengths of natural bone. Applications of tissue engineering and 3D bone printing are beginning to unravel the enigma of bone formation and provide new solutions to regenerate bone tissue.
Due to numerous advantages, bioresorbable materials, such as beta-tricalcium phosphate (β-TCP), are gaining traction in orthopedics and maxillofacial surgery to restore bone loss over titanium and autografts. Researchers and clinicians are experimenting, discovering, and achieving success with 3D-printed ceramic scaffolds and personalized single-point-of-care implants.
β-TCP scaffolds support Schwann cell growth and behavior
A key challenge of bone tissue regeneration when using ceramic scaffolds is ensuring sufficient vascularization (Khayatan et al., 2024, Abdullah et al., 2024). While many strategies are being researched and developed in vitro, one promising avenue is facilitating the incorporation of nervous system glia, particularly Schwann cells (SCs).
Why is research focusing on Schwann cells?
Throughout the body, nerves and blood vessels typically run in parallel, suggesting a natural synergy that can be harnessed for improved vascularization in 3D-printed scaffolds in vitro and ultimately an implant in vivo.
Schwann cells, the myelinating cells of the peripheral nervous system, are known not only for their role in peripheral nerve repair and providing innervation to traditional grafts but also for their significant angiogenic potential through growth factor secretion. SCs wrap around axons to improve signal conduction and assist in neural regeneration after injury, showcasing their versatility and regenerative capabilities.
The geometry of 3D-printed scaffold influences cell growth
Research demonstrates that the geometry of a 3D-printed scaffold can affect the functional performance of the SCs. Instead of random, patterned porous scaffolds can induce multiple beneficial behaviors, including cellular alignment, migration, and effectiveness at directing and inducing axonal ingrowth (Sweet et al., 2015).
What may appear as an incremental step in 3D printed scaffold design can ultimately shape success when incorporated into 3D bone-printed implants for bone loss treatment. As shown in Figure 1, SCs grew and proliferated well on the scaffolds which represent a more realistic 3D micro-environment compared to a culture plate, and furthermore showed enhanced growth with the patterned porosity.
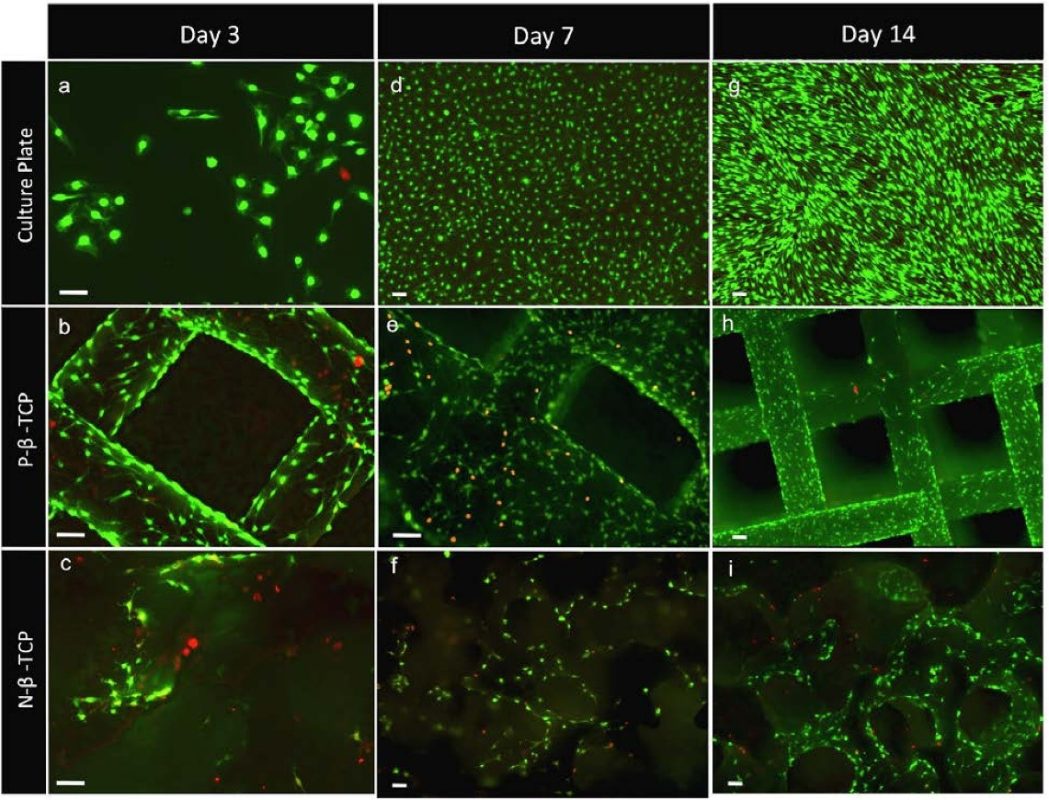
Figure 1: Staining highlights the pattern of clustered growth on the scaffolds (e, f, h, i) cultured, non-patterned and patterned scaffolds. Reposted according to the Creative Commons Attribution License from Sweet, L., et al. (2015). Geometrical versus random β-TCP scaffolds: Exploring the effects on Schwann cell growth and behavior. PLoS One, 10(10), e0139820.
β-TCP scaffold concentration influences natural bone formation
In a most recent study based on an ectopic mouse model, higher content of β‐TCP in ceramic scaffolds significantly impacts the ability of hBMSCs (human bone marrow-derived mesenchymal stem cells) to support angiogenesis and promote mature osteogenesis (Pereira et al., 2019). Two concentrations of hydroxyapatite (HA) and β‐tricalcium phosphate (β‐TCP) were tested in ratios of 60/40 and 20/80.
Results show that the 3D-printed scaffolds with higher β‐TCP content induced faster and more extensive vascular growth and mature bone deposition compared to those with lower β‐TCP content (Pereira et al., 2019). The researchers report that the in vitro culture model had a higher rate of released calcium ions from the calcium phosphate. This, in turn, enhances VEGF (vascular endothelial growth factor) secretion in hBMSCs and stimulates endothelial cells to form capillaries.
These findings demonstrate the influence of bioceramic scaffold composition on bone formation and maintenance. Further, the findings represent another parameter researchers can explore to pave the way for unravelling solutions for specific diseases, conditions and injuries.
Personalized point-of-care β-TCP implants
When research continues to build foundational insights, such as patterned scaffold designs and higher β‐TCP compositions, orthopedics and oral and maxillofacial surgeons can take advantage of a longer runway to test personalized point-of-care implants (Maintz et al., 2024). A recent case report revealed how powerful computer-aided design and manufacturing (CAD/CAM) can be when paired with 3D bone printing (Schönegg et al., 2024).
The case report presented a 63-year-old female patient with significant bone loss who was treated with a custom β-TCP implant to regenerate lost bone before receiving a dental implant. In this case, the 3D-printed β-TCP implant was a pre-implant to restore the height and width of a large defect in her alveolar ridge. Based on the patient’s cone-beam computed tomography (CBCT) and intraoral scan, a digitally designed 3D bone-printed implant was fabricated.
At nine months, the custom β-TCP implant successfully integrated into the surrounding bone, allowing dental implant placement. Two years later, the patient demonstrated excellent oral and peri-implant health (Schönegg et al., 2024).
Leveraging 3D printed β-TCP scaffolds in research
Researchers can leverage and experiment with scaffold compositions based on bioceramics using Ossiform’s β-TCP P3D scaffolds. β-TCP bioceramics historically are known to offer several properties to promote bone tissue regeneration and remodeling (Khayatan et al., 2024):
- Biocompatibility: implantable without adverse reactions
- Osteoconductivity: facilitates bone regeneration
- Bioactivity: promotes interactions with the natural environment
- Mechanical strength: ensures durability and stability
- Porosity: allows for infiltration of cells and fluids
- Resorbability: gradually replaced by natural bone
- Thermal stability: maintains integrity in physiological temperature
Indeed, as evidence, researchers are putting these properties to the test in 3D environments, extending knowledge from the Petri dishes to mouse models and ultimately to single-point-of-care in patients.
References
- Abdollahi, F., Saghatchi, M., Paryab, A., Malek Khachatourian, A., Stephens, E. D., Toprak, M. S., & Badv, M. (2024). Angiogenesis in bone tissue engineering via ceramic scaffolds: A review of concepts and recent advancements. Biomaterials Advances, 159, 213828.
- Huang, B., Yang, M., Kou, Y., & Jiang, B. (2024). Absorbable implants in sport medicine and arthroscopic surgery: A narrative review of recent development. Bioactive Materials, 31, 272-283.
- Khayatan D, Bagherzadeh Oskouei A, Alam M, et al. Cross Talk Between Cells and the Current Bioceramics in Bone Regeneration: A Comprehensive Review. Cell Transplantation. 2024;33. doi:10.1177/09636897241236030
- Maintz, M., Tourbier, C., de Wild, M., Cattin, P. C., Beyer, M., Seiler, D., … & Thieringer, F. M. (2024). Patient-specific implants made of 3D printed bioresorbable polymers at the point-of-care: material, technology, and scope of surgical application. 3D Printing in Medicine, 10(1), 13.
- Pereira, R. C., Benelli, R., Canciani, B., Scaranari, M., Daculsi, G., Cancedda, R., & Gentili, C. (2019). Beta‐tricalcium phosphate ceramic triggers fast and robust bone formation by human mesenchymal stem cells. Journal of tissue engineering and regenerative medicine, 13(6), 1007-1018.
- Schönegg, D., Essig, H., Al-Haj Husain, A., Weber, F. E., & Valdec, S. (2024). Patient-specific beta-tricalcium phosphate scaffold for customized alveolar ridge augmentation: a case report: Case Report: patient-specific β-TCP scaffold for alveolar ridge CBR. International Journal of Implant Dentistry, 10(1), 21.
- Sweet, L., Kang, Y., Czisch, C., Witek, L., Shi, Y., Smay, J., … & Yang, Y. (2015). Geometrical versus random β-TCP scaffolds: Exploring the effects on Schwann cell growth and behavior. PLoS One, 10(10), e0139820.